Novel Amino Acids Come of Age
By Jason Socrates
Bardi
Some time in the few hundred million years between the formation
of earth's crust and the emergence of earth's oldest known
cells, cyanobacteria, protein synthesis evolved. Whether it
evolved in cyanobacteria 3.5 billion years ago or in some
earlier precursor decades before is unknown. But we do know
that the proteins were synthesized using only 20 amino acids
because all higher organisms that have come after have used
the same 20.
"Why do all forms of life we know use the same common 20
amino acids and only 20 (seleno cysteine excluded)?" asks
Peter Schultz, professor of chemistry and Scripps Family Chair
of The Skaggs Institute of Chemical Biology. "Can we change
this by adding new amino acids to the genetic code and can
we use these new amino acids to change the structures and
functions of proteins in interesting ways?"
The answer to his last three questions is now, apparently,
yes.
About 15 years ago, when Schultz was at the University of
California at Berkeley, he began inserting novel amino acids
into proteins in vitro, and about five years ago, he
began working on a project to do it in vivo. "We are
interested in proteins because, as chemists, the most fascinating
class of molecules are proteins, which have functions ranging
from photosynthesis to signal transduction to gene regulation."
says Schultz.
"And," he adds, "when chemists look at molecules, they say,
'How can we better understand how they work, and how can we
rationally manipulate their structure to create interesting
new functions?'"
Schultz reckoned that it would be possible to enhance the
chemical, physical, and biological properties of proteins
by adding novel amino acidsones that are not among the
20 that all living organisms use. He wanted a way to do this
easily and in vivo, because direct chemical or biochemical
synthesis of a protein containing unusual amino acids, while
possible, is limited, laborious, and low-yielding.
"To make proteins in a robust way, one has to do it inside
cellsit's difficult to synthesize proteins in a test
tube," says Schultz.
Success Story
Schultz, his student Lei Wang, and their colleagues announced
in a Science paper earlier this year that they had
succeeded in adding the new amino acid O-methyl-tyrosine to
the genetic code of E. coli. O-methyl-tyrosine is the
first of several new amino acids they are working on site-specifically
inserting in vivoa proof of principle.
Also called "unnatural" because they are not among nature's
original 20, these novel amino acids have the same carboxyamino
backbone as the 20 standard amino acids but different side
chains. Some have just slightly altered chemical structures
and others have new functional groups added. In proteins,
these differences may alter everything from structure and
folding to activities. Certain "designer" side chains may
even impart novel functionality.
With his new methodology, Schultz believes it will not be
long before this technology is here. "It should be possible
to put in a fluorescent probe or a photoaffinity label at
any site on any protein in the cell," says Schultz.
The idiom, "You can't teach an old dog new tricks" doesn't
even come close to describing how difficult it should have
been to make organisms incorporate novel amino acids into
their protein chains. After all, every organism in nature
has been using the same 20 amino acids since the primordial
soup. Organisms have to maintain fidelity in replication,
and so life has evolved myriad mechanical ways of making sure
only those 20 amino acids get incorporated into proteins.
Schultz and his colleagues did it anyway.
Working the Code
When a protein is expressed, an enzyme reads the DNA bases
of a gene (A, G, C, and T), and transcribes them into RNA
(A, G, C, and U). This so-called "messenger RNA" is translated
by another proteinRNA complex, called the ribosome,
into a protein. The ribosome requires the help of transfer
RNA molecules (tRNA) that have been "loaded" with an amino
acid. Protein specificity comes from the fact that the tRNA
recognizes only one codon and gets loaded with only the one
amino acid that is specific for that codon.
For every codon on the mRNAevery three basesthe
ribosome attaches another amino acid to the chain. But even
though there are 4x4x4 = 64 different codons (UAG, ACG, UTC,
etc.) there are only 20 amino acids that all organisms use
to produce proteins. Some of the 64 codons are redundant,
with several coding for the same amino acid, and three of
them are nonsense codonsthey don't code for any amino
acids.
These nonsense codons are useful because normally when a
ribosome that is synthesizing a protein reaches a codon that
does not code for any tRNA, the ribosome dissociates and synthesis
stops. Hence nonsense codons are also referred to as "stop"
codons. One of these, called the amber stop codon, UAG, played
an important role in Schultz's research.
If the cell is provided with an amber suppressora
tRNA that recognizes UAGthen the ribosome will grow
the chain with the amino acid that this new tRNA carries and
synthesis does not stop. Any codon in an mRNA that is switched
to UAG will carry the new amino acid in that place and the
ribosome will resume reading the full-length message.
Site-directed mutagenesis is an application of amber suppression,
and by simple extension, a tRNA that recognizes UAG and carries
a novel amino acid could be used to site-specifically incorporate
novel amino acids. If he could create an amber suppressor
tRNA that carried a novel amino acid and an enzyme to load
the amino acid on that tRNA, Schultz knew he would have the
ability to site specifically add the novel amino acid to a
growing protein chain wherever he inserted the codon UAG.
All he needed was a tRNA unique to that codon and a synthetase
"loading" enzyme to load the unnatural amino acid on that
tRNA. Then he would have a simple and robust way to put a
completely original residue anywhere he wanted in a protein.
99.99 Percent Fidelity
This was not easy. "It's hard to find synthetases that recognize
novel amino acids because nature has designed them to recognize
only the natural ones," says Research Associate Steve Santoro,
a TSRI graduate. And overcoming that obstacle, there is also
the requirement that the new tRNA/synthetase pair be functionally
orthogonal, meaning the new pair must not interact with any
of the existing tRNAs or synthetases.
"The new tRNA should not be aminoacylated by any existing
synthetase, and likewise, the new synthetase should not recognize
any existing tRNA," says Wang, who has been working on the
project from its early beginnings. "There should be no crosstalk."
The orthogonal synthetase had to be engineered so that it
charged the orthogonal tRNA with an unnatural amino acid but
not any natural amino acids and do so with a fidelity that
is as high as normal99.9 percent or highersomething
that proved difficult.
"That was the goal," says Schultz, "though we thought we
wouldn't get there in ten years."
1 | 2 |
|
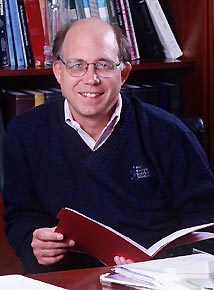
"Historically, chemists have been interested
in synthesizing molecules," says Professor Peter Schultz.
"We are interested in synthesizing function." Photo
by Biomedical Graphics.
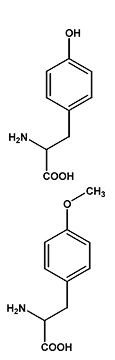
The chemical structures of the amino
acid tyrosine (top) and the novel amino acid O-methyl-tyrosine
(bottom).
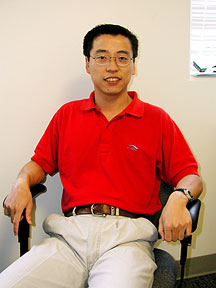
"You just need to add the novel amino
acid to the culture and grow the cells," says graduate student
Lei Wang. Photo by Jason
S. Bardi.
|