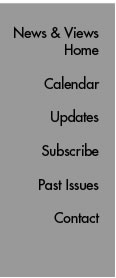 |
 |
Researchers Observe Single Protein Dimers Wavering Between Two Symmetrically Opposed Structures
By Renee Twombly
Researchers at The Scripps Research Institute, the University of California, San Diego, and Ohio State University have used a very sensitive fluorescence technique to find that a bacterial protein thought to exist in one "natural" three-dimensional structure (shape), can actually twist itself into a second form, depending on the protein's chemical environment. One folded form is active and the other is inactive, but the protein can easily morph from one state to another.
The scientists say their discovery, published in the online Early Edition of in The Proceedings of the National Academy of Sciences (PNAS) on June 8, 2009, emphasizes that such shape shifting by proteins is a common way of regulating cellular activities. This direct view provides a neat example of the dynamic and complex nature of proteins, contrasting with a single preferred folded structure that is determined by the energy balance between the amino acids that make them up.
The group of Scripps Research Associate Professor Ashok Deniz has conducted a series of studies that use novel fluorescence methods to show that both simple and complex proteins can swiftly change their structures. In March, Deniz and his Scripps Research team published a study, also in PNAS, demonstrating how a simple protein associated with development of Parkinson's disease can switch shapes back and forth between different structures, depending on its binding to molecular partners.
Now, a collaboration among the Deniz laboratory, the group of José N. Onuchic of the University of California, San Diego, (UCSD) and the laboratory of Thomas J. Magliery of Ohio State University, has shown that a protein dimer made up of two identical parts can actually twist itself so that one copy of the protein is turned upside down if its environment is only slightly altered.
"It has long been a puzzle as to how proteins, which can theoretically adopt an extremely large number of structures even with a single chain of amino acids, end up folding themselves to a well defined three-dimensional structural basin," says Deniz. "While the concept of a funneled energy landscape explains folding, protein function often requires more than one stable three-dimensional shape. Theoretical work generalizing energy landscape theory combined with these experiments shed light on how symmetry can result in proteins being able to populate structures that are dramatically different, a feature very important in cellular regulation and function."
A deeper understanding of how living systems use protein shape shifting to control cellular processes would allow better design of therapeutics that can alter these shapes in order to turn proteins on and off and control disease, Deniz notes.
Head or Tail?
In the new study, the researchers examined a protein known as Rop (repressor of primer), expressed in E. coli bacteria, which binds to RNA to regulate the number of copies of plasmid genes produced within the bacteria. This small "homodimer" is made up of two identical hairpin coils of amino acids, and has often been used as a model system to study protein structure and stability.
The protein has been found in two different conformations: the "anti" wild type (natural) conformation occurs when the "head" of one hairpin is paired with the "tail" of the second, and the "syn" structure is made up of the two bound head-to-head. The anti structure is thought to be the active conformation that binds to RNA, while the syn structure—found in a mutant—is inactive.
But theorist Onuchic and his coworkers had proposed that there is a delicate balance of energy for the syn and anti structures, with mutations or conditions being able to alter this balance. They predicted that certain mutations in the protein sequence would allow a single sequence to populate both structures, which they felt might explain puzzling features of the stability and folding speed of these molecules.
To test if this was true, Deniz and his group at Scripps Research used single-molecule fluorescence resonance energy transfer (smFRET), a tool they have helped develop. This technique, which Deniz calls a "molecular ruler," measures light emitted from two fluorescent dyes that are attached to amino acids in the protein. The color of the measured light provides information about molecular distances that reveals a protein's structure. Observing structures of individual proteins or complexes is an advance over typical ways of measuring complex and dynamic protein structures, which often end up losing information by averaging data over a large number of them, Deniz says.
The scientists studied three different Rop proteins—the wild type anti, the mutant syn, and another mutant known as A2L2. They found that although the A2L2 was active, it surprisingly configured itself mostly in the syn structure, which was believed to be inactive.
The scientists then altered the protein's environment by adding a little "denaturing" chemical, which helps destabilize the protein structure. They found that as more of this chemical was added, the A2L2 proteins increasingly switched structure from mostly syn to significantly anti by twisting one of the protein copies in the dimer by 180 degrees. Additionally, by using a more complex experiment with an additional differently-colored fluorescent dye, they discovered another surprise. The dimers were able to switch structures without separation of the constituent copies, even though the structure change was very drastic.
"We were able to easily switch A2L2 from an inactive state to one that could bind RNA, and it takes only quite mild changes in conditions to make this change. These direct observations show that this protein dimer has two native structures available to it, and that minor perturbations can cause this balance to be altered," Deniz says. This suggests that cells could easily regulate how such proteins within them are folded, and thus alter their function.
Theoretically, it would only take different binding partners or changes in the cell's pH, ions, or the presence of other small molecules to regulate how a protein is folded within a cell, he says. "Furthermore, proteins could be folded in one structure in one area of the cell, and configured differently in another location of the same cell depending on whether or not they need to be active."
"Such a powerful mechanism has lots of clinical implications," Deniz says. "Small mutations in proteins could throw off the delicate balance of energy that is needed between conformational structures of proteins in a cell, leading to disease. If so, a drug might be able to reverse those kinds of imbalances."
In addition to Deniz, Onuchic, and Magliery, authors of the paper, titled "Direct single-molecule observation of a protein living in two opposed native structures," are first authors Yann Gambin of Scripps Research and Alexander Schug of UCSD, as well as Edward A. Lemke and Allan Chris M. Ferreon of Scripps Research, and Jason J. Lavinder of Ohio State University.
The study was funded by grants from the National Institutes of Health and the National Science Foundation.
Send comments to: mikaono[at]scripps.edu
|
 |
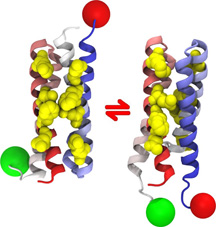
New research shows that a protein dimer called Rop, which is made up of two identical parts, can actually twist itself so that one copy of the protein is turned upside down if its environment is only slightly altered. Such shape shifting by proteins may be a common way of regulating cellular activities.
|
 |