Retro Synthetic Analysis, or Is Nature Perfect?
The
gods of the earth and sea
Sought through nature to find this tree,
But their search was all in vain:
There grows one in the human Brain.
From
The Poems of William Blake
By Jason Socrates Bardi
Finding chemicals with biologically useful properties has
become the stuff of modern day legends. Compounds that exhibit
anti-tumor activity, viral replication inhibitor molecules,
thermally stable enzymes, and other holy grails that achieve
biological goals have been found in nature.
And so from the Pacific yew groves to the deep sea vents,
we quest after these undiscovered, potentially useful compounds.
Any tree, toad, or random gram of terra firma could contain
the next ibuprophen or AZT. Once found, these compounds can
be studied, solved, synthesized, and mass producedto
the benefit of all mankind.
Nature provides and science supplies.
But uncovering natures secret compounds and finding
ways to synthesize them is only the beginning. A basic science
laboratory is interested in not only what we can get from
nature, but what we can learn from it.
There is this impression that if it comes from nature,
then we cant do any better, says Dale Boger, who
is the Richard and Alice Cramer Professor of Chemistry at
The Scripps Research Institute (TSRI). "But in fact, nature
rarely makes the molecules for the reasons that we find them
useful or interesting."
Because the compounds we discover may not have evolved to
do exactly what we want them to do, we cannot expect to find
the best agents in nature. However, what we can expect to
find are lead compounds from which we can gain insight into
the design of others.
Boger calls this "constructionist science"the synthesis
of function, not solely the synthesis of molecules. And he
and his colleagues at TSRI seek to use the tools of organic
synthesis to identify, imitate, understand, exploit, and sometimes
surpass what nature provides.
A Problem in Search of its Chemistry
The science starts, of course, with synthesizing compounds
that exist in nature. "Were a group that chooses its
synthetic targets based on the properties of the molecules,"
Boger says, "And a large proportion of our targets have a
unique mechanism or properties associated with them that make
them interesting in their own right."
Boger and his colleagues use the technique known as retro
synthetic analysis, where a scientist looks at a structure,
moves back one step to a precursor of this structure, and
then thinks of a way to convert that precursor to the final
product. There are usually multiple possible precursors, and
each of these will have several precursors that could be used
to form them.
A path must be chosen, and the selection usually reflects
the personality, expertise, and the interests of the chemist.
"If you have a hundred chemists, youll have a hundred
different routes to the final molecule," says Boger.
After synthesizing an interesting natural product, further
investigations can show what it is about the compound and
its interaction with its biological target that makes it active,
and this information can then be used to make simpler or better
agents.
This type of work is time-consuming. A novel synthesis may
require as many as 40 steps, each step being a reaction in
which a molecule a little closer to the target is formed.
Some steps may be easy to anticipate beforehand, and others
may have to be invented from scratch, and each step may require
10 to 20 novel approaches before finding one that works well.
Each approach may have to be repeated several times, and each
time various analytical tools have to be used to determine
the reaction product and yield. Then there may be 10 to 20
optimizations at each step as well.
Boger estimates his laboratory spends about 90 percent of
its time tackling various syntheses. And a project may take
several years from start to finish.
"Its not something that you can make today and analyze
tomorrow," says Boger.
1 | 2 |
|
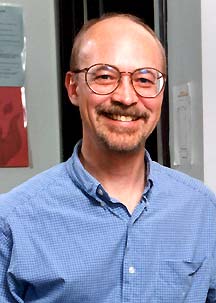
There are structures that we are
working on today that 10 or 12 years ago would not have been
considered realistic to prepare in the laboratory. And other
things you can do today with 10, 20, or 1,000 times less material
than it would have taken a decade ago.
|