(Page 2 of 2)
Uncharted Waters
Bacteria use a host of methods to foil antibiotics. The bacterium
S. aureus produces an enzyme, ß-lactamase, which
specifically degrades penicillin and its analogues through hydrolytic
cleavage of the penicillin ß-lactam ring. This "target
modification" mechanism is also used by S. aureus and other
bacteria against different classes of antibiotics, such erythromycin
and chloramphenicol. There are a host of other mechanisms bacteria
employ to evade antibiotics, such as encoding enzymes that sequester
antibiotics by binding to them, undergoing small point mutations
in the molecular targets that lower a drugs affinity,
overproducing a drugs substrate in the cell, and efflux
pumps.
Efflux pumps and transporters are perhaps two of the most
difficult structures to study, though, becauselike all
other membrane proteinsthey are notoriously hard to
solve. Less than one half of one percent of the structures
contained in the Brookhaven National Laboratory Protein Data
Bank are of integral membrane proteins, despite the fact that
over a third of all proteins in the body are in the membrane.
The difficulty with solving membrane proteins begins with
obtaining them. Producing enough protein to work with can
be insurmountable. A crystallographer might need several milligrams
of protein to start with, but since most channels and transporters
constitute such a small percentage of the cellular composition,
getting enough material to work with becomes a challenge.
"You just cant grow that many cells," says Chang, who
adds that all the membrane structures solved before 1998 are
from naturally abundant sources.
Even assuming success in producing sufficient quantities,
the proteins must be purified in their native state, which
entails purifying them in the presence of detergents so that
their hydrophobic membrane-spanning region can be surrounded
by the hydrophobic ends of detergent molecules in micelle-like
formations. The conditions are highly sensitive to such variables
as detergent type, concentration, pH, and ionic strength.
Worse, these conditions are almost always unique, demanding
a lengthy trial and error search of an unknown biochemical
landscape for that exact novel solution in which the protein-detergent
complexes will be soluble and stable. "You have to draw on
your own experience," says Chang, "You have to have quite
a lot of it, actually."
Then a whole separate set of novel conditions must be worked
out for growing the crystals, described by Chang as, "crystallizing
out of soap." Several tricks can be employed, such as using
antibody fragments, cubic lipid phases, or non-detergent systems.
But, says Chang, these techniques are highly system specific
and may only work with one or two proteins. Crystallizing
presents another large problem in that the costs of the detergents
can easily add up to hundreds of thousands of dollars.
Finally, any crystals that are grown can be difficult to
work with because of their solvent content. Most water-soluble
protein crystals have a protein-solvent ratio as high as 3:4almost
as hard or as dense as salt crystals. Membrane protein crystals,
however, have a much higher solvent content because of the
added bulk of the detergent micelles. As much as 88 percent
of the membrane protein crystal may be a mixture of solvent
and detergent, which makes the crystals unusually fragile
under an x-ray beam. Chang calls it "shooting through Jell-O."
Every part of the process must come together for the science
to work. No protein, no crystals; no crystals, no structure.
Changs solution follows Thomas Edisonstry
as many different targets and conditions as necessary, build
on these experiences, and go with those that work. "Its
really still a new field," says Chang. "Theres almost
no literature on how to crystallize membrane proteins."
Once he has crystals, Chang will then make data collection
trips to synchrotrons at the Stanford Synchrotron Radiation
Laboratory and the Advanced Light Source at UC Berkeley. Synchrotrons
are radiation sources that use x-ray radiation produced by
electrons moving close to the speed of light in particle accelerator
storage rings. These x-rays are intense, collimated, and tunable
to various wavelengths, which make synchrotrons particularly
useful for examining protein crystals. Chang, for example,
will bombard his frozen membrane protein crystals with synchrotron
x-rays and collect diffraction patterns for a variety of crystals.
He will spend months analyzing and refining this data, and
eventuallyhopefullysolve a novel structure of
a membrane complex involved in antibiotic and chemotherapy
drug resistance.
Chang Honored at White House
Chang came to TSRI after spending three years as a postdoctoral
fellow in Douglas Reess laboratory at the California Institute
of Technology. In October, just over a year after he arrived,
Chang was named one of the recipients of the Fifth Annual Presidential
Early Career Awards for Scientists and Engineers, the highest
honor bestowed by the United States government on young professionals
at the outset of their careers. He spent a day in Washington,
D.C., touring the National Institutes of Health and standing
for a presentation ceremony at the White House.
Chang takes his award out of a file in his desk drawer and
shows it to mea simple design with nice lettering and
William Jefferson Clintons bold signature across the
bottom. It is still in the presidential blue folder it came
in, and after a few moments, he flips the cover closed and
slips it back in the file drawer. "I havent had time
to get it framed," he explains.
1 | 2 |
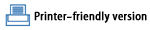
|
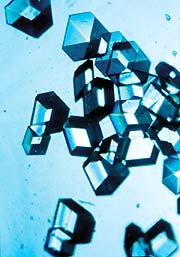
Protein crystals, such as those of insulin pictured
here, are notoriously difficult to make. "You have to draw
on your own experience," says Chang. "You have to have quite
a lot of it, actually." (Photo courtesy of NASA).
|