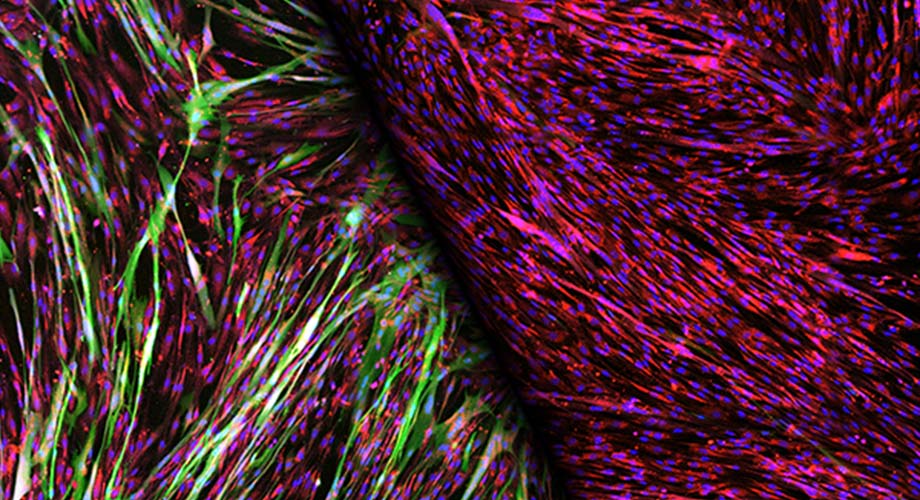
Stained muscle cells reveal robust gene delivery via a gene therapy vector modified to bind to insulin receptors, left (green). The vibrant photo became the December cover image for the scientific journal Molecular Therapy Methods and Clinical Development. (Image courtesy of Cody Jackson, Scripps Research)
Targeting muscle cells’ insulin receptors allows more efficient gene therapy
Method could allow significant dosage reduction, addressing key concerns, scientists find.
December 30, 2020
JUPITER, FL—The potential for gene therapy to address genetic diseases is immense, but several challenges have held up progress. Improving the efficiency of the gene delivery system could be a viable way to resolve many of them, says Scripps Research, Florida virologist Hyeryun Choe, PhD.
In a paper published in the open access journal Molecular Therapy: Methods & Clinical Development, Choe and colleagues report a more productive method of introducing therapeutic genes: by programming the gene therapy vector to seek out muscle cells, via abundant insulin receptors displayed on the surface of those cells.
The most-studied method of gene therapy has issues of excessive cost and high dose-associated toxicity risks, Choe explains. Lowering the dose needed to bring about the desired treatment should ease both problems, she explains. To accomplish this, Choe and graduate trainee Cody Jackson sought to engineer a viral gene delivery system to target muscle cells. They reasoned that muscle cells’ longevity, among other traits, would make them the best cells for the job.
“Muscle cells don’t divide, so you have them for your whole life,” Choe says.
One of the ways scientists administer therapeutic genes is by engineering viruses to deliver them into cells. They start with a benign virus, such as the adeno-associated virus, or AAV, which doesn’t replicate in humans, and reengineer its genome to carry the genetic payload needed to treat or prevent disease.
The virus can be altered to display a protein fragment perfectly structured to bind to a desired cell receptor, so that it unlocks entry to a specific cell type, like a key made for one specific type of lock. The next challenge Choe’s team faced was to identify the most promising receptor on muscle cells, and find the right “peptide” or protein fragment, that would bind tightly to that receptor.
The protein fragment that Choe’s team identified recognizes muscle cells’ insulin receptors with high affinity, explains Jackson. They found the introduction of that insulin-mimetic peptide boosted the efficiency of vector uptake by nearly twentyfold compared to standard methods, thus allowing a significantly lower dose of the therapeutic virus to deliver a gene of interest. It showed that muscle cells are very good targets for gene therapy, says Jackson.
“Something unique about muscle tissue is its capillary network. It allows the proteins produced there to easily diffuse into the blood,” Jackson explains. “This platform could be used for any disease where you want the protein to be circulating throughout the body, systemically.”
They checked whether the use of a vector targeting insulin receptor could impact the blood sugar of treated animals. Testing proved it did not, he adds.
The U.S. Food and Drug Administration has so far approved gene therapies for an eye condition that causes congenital blindness, and a genetic disease called spinal muscular atrophy. The treatments are life-changing, but can cost between $500,000 and $2 million per patient.
“With enhanced efficiency, doses can be lowered, and with lowered doses, costs and toxicity-related adverse events can be reduced,” Choe says.
Choe says the insulin receptor-targeting strategy is useful for another reason. Because the insulin receptor they targeted is conserved across multiple animal species, the potential new therapies can easily be evaluated in laboratory animals.
It’s clear that targeting muscle cells via insulin receptors represents an important improvement in gene therapy technology, and offers potential routes to address both genetic and infectious diseases, such as HIV, for which neutralizing antibodies are often produced via an intramuscular delivery of an AAV vector, says Choe.
“This approach worked like a charm,” she says.
Jackson’s work on the study earned him the cover image for the scientific journal. Conducted as part of his PhD thesis project, it also helped him become the first student to complete his doctorate through the joint MD/PhD program offered cooperatively by the Scripps Research, Florida branch of the Skaggs Graduate School of Chemical and Biological Sciences, and Florida Atlantic University’s Charles E. Schmidt College of Medicine.
The rigorous joint-degree program demands real commitment, Jackson acknowledged. It requires a bachelor’s degree plus two years of medical school coursework, followed by four to five years of doctoral studies in Scripps Research labs. But that’s not the end of it. Another two years of clinical rotations are required to earn the white coat and the letters MD. He’ll start his clinical rotations this spring.
“I am hoping that completing the training in both programs means the opportunities will be open for me to do clinical practice, or research, or both,” Jackson says.
Skaggs Graduate School Associate Dean Christoph Rader, PhD, praised Jackson’s hard work and impact.
"We were fortunate to recruit Cody Jackson to our joint MD/PhD program. His dedication and drive have been outstanding,” Rader says. “As our first graduate, he will always be remembered."
In addition to Jackson and Choe, the study’s authors include Michael Farzan, Audrey Richard and Amrita Ojha of Scripps Research; Kimberly Conkright, Jeffrey Trimarchi, Charles Bailey and Michael Alpert of Emmune, Inc.; and Mark Kay of Stanford University.
This study was supported by grants from the National Institutes of Health, including R37 AI091476, R44 AI134269 and R44 TR003501.
For more information, contact press@scripps.edu