Of Molecules and Methods
By Ulrika Kahl
Most people use tennis balls when they, well, play tennis.
In addition to using them to play tennis, Professor Julius
Rebek, director of The Skaggs Institute for Chemical Biology
at The Scripps Research Institute (TSRI), uses tennis ballsand
softballs, jelly donuts, and other itemsto illustrate
the shape of, and forces within, the molecular structures
that he and his colleagues study in the laboratory.
"It's all about the space," says Rebek, explaining why molecules
behave in the way they do. Parameters like temperature, pH,
and illumination of course matter, but space is critical.
Molecules want to fit into the space, and make sure that they
have enough of it, although not too much of it either. And,
according to Rebek, the magic number is 55. "If you have a
space, and you fill 55 percent of it, then you have a happy
complex," he explains. Organic solutions, for instance, are
55 percent molecules, the rest pure space. Typical crystals
are 75 percent molecule, 25 percent space.
Rebek uses a tennis ball to illustrate his point. If you
cut a tennis ball along the curved line, you will end up with
two identical halves. Imagine that these halves are molecules.
In the space between these two large host molecules, other
molecules, like methane and similar gases, can be encapsulated.
The tennis ball capsule and the encapsulated molecule will
assemble into a complex, held together by reversible intermolecular
hydrogen bonds. If the encapsulated molecule fills 55 percent
of the space in the capsule, the whole complex will be in
equilibrium, "a happy complex."
Molecules such as benzene, whose structure is flat, will
need a different environment. Using another of Rebek's examples,
benzene would be better off in the space of a jelly donut.
But benzene, too, will still strive towards 55 percent occupancy.
Rebek and his colleagues presented their tennis ball capsules
in 1994 in an article in Science. There, they showed that
the capsule molecules they had created in a chemical reaction
could form homodimers (a complex consisting of two identical
molecules) that assembled spontaneously, resulting in an energy-minimized
structure. In the sphere inside the dimer capsule, small molecules
like methane, ethane, and ethylene could be entrapped reversibly,
as was shown with nuclear magnetic resonance (NMR). The degree
of occupancy was shown to be largely determined by the nature
of the solvent the complex was in. The solvent molecules would
compete with the guest molecules for the space inside the
capsule, and the molecule coming closest to filling 55 percent
of the space would be the one most likely to be found in the
capsule.
From the perspective of a chemist, Rebek's findings lead
to an elegant molecular assembly technique. Instead of having
to rely on extreme conditions or complicated chemical syntheses,
molecules recognize each other and self-assemble in solution.
This process is strikingly similar to that used by living
cells, which may have taken millions of years to refine to
perfection. Complex cellular structures like membranes, ribosomes,
and viruses are all products of self-assembling mechanisms.
From a medical perspective, this encapsulation strategy
holds promise for creating a technique using molecular shells
to deliver drugs to target cellsin fact, in a manner
similar to that which viruses use, entering host cells and
releasing their DNA inside. It also suggests guidelines for
designing drugs. "If you have a structure of an enzyme and
want to design an inhibitor," says Rebek, "then look for something
that fills 55 percent of the binding site." In other words,
you will have the greatest chance of succeeding if you give
the drug an optimal amount of space to perform its action.
The self-assembly of molecules that Rebek and co-workers
observed in their study has become a focus of further research
in the past half-decade. Self-assembly can be used for the
rapid construction of large and elaborate molecular structures,
an approach that is widely used in what is referred to as
combinatorial chemistry. Combinatorial chemistry is a method
for rapidly making a large number of different molecules in
one and the same reaction, and is currently one important
part of Rebek's research.
"Seven years ago, no companies were interested in combinatorial
chemistry," Rebek says. "Today, every drug company has a combinatorial
chemistry section. Using combinatorial chemistry is a smart,
efficient way to study molecular interactions and develop
novel drugs."
Combinatorial libraries of molecules rely on a numbers game.
Let's say a scientist is presented with a core molecule with
four reactive corners. If he or she throws in 20 bases, for
example amines, which are all able to react with each one
of the four reactive groups of the core molecule, in 10 minutes
the reaction will yield around 100,000 statistically possible
compounds. Then, if the researcher runs this library of compounds
in an activity assayfor instance one in which the inhibition
of the protease trypsin is measuredand get a positive
signal, it means that one or more compounds in the library
are potential competitive protease inhibitors, acting on trypsin.
The most problematic step in combinatorial chemistry is
the analysis of the libraries obtained. It iseven with
the most advanced instruments and analytical methods availablehard
to separate and characterize hundreds of thousands of molecules
in a reaction mix. There are two main approaches to creating
combinatorial libraries: on solid support and in solution.
The latter method is the one Rebek prefers. To identify the
products in a solution-created library, one can either use
tagged molecules in the reaction, or employ the method of
deconvolution.
Deconvolution is an iterative selection procedure, in which
several smaller sublibraries are first created, each of them
lacking a few of the initial building blocks. Activity measurements
of the sublibraries will then reveal which of the building
blocks did not contribute to the overall activity in the main
library. Another set of sublibraries is then made based on
the first ones, and the activity is once again measured. Eventually,
after the cycle is repeated a number of times, only one or
a few active compounds are left. These compounds can be separated
with chromatography methods like high-performance liquid chromatography
(HPLC). Finally, mass spectrophotometry and NMR analysis will
reveal the composition and structure of the active compounds.
The unique efficiency and complexity of combinatorial chemistry
becomes more useful as we learn more about the mechanisms
that keep the cells and organs in our body running. For instance,
based on the known structure of the binding site for a certain
nerve transmitter in the brain, a researcher may be able to
choose potential building blocks with high precision in the
combinatorial synthesis of a drug targeted at this binding
site.
Rebek's most important contributions to science to date
are probably the many experimental methods that he together
with co-workers developed in self-assembling systems and combinatorial
chemistry over the years. These accomplishments have grown
out of Rebek's love of designing and building molecules and
methods.
Rebek has been drawn to designing and building throughout
his life. Initially, he planned to become an architect. The
architect dream was, at least to some extent, fulfilled by
a certain Cape Cod beach housedesigned by Rebek, of
course.
When Rebek came to TSRI in 1996, he became director of the
newly founded Skaggs Institute for Chemical Biology, which
was funded by a generous gift from Aline and Sam Skaggs through
the Skaggs Institute for Research and their family foundation,
the ALSAM Foundation. Rebek appreciates the commitment of
Skaggs family members, who frequently visit TSRI, and show
an interest in the research they have made possible.
In his role as director of the Skaggs Institute and head
of an active laboratory, Rebek has had the opportunity to
build both molecules and a future generation of scientists.
Throughout his career, over 150 students and associates have
studied under Rebek's guidance. Along with his contributions
to science, Rebek's investment in future scientists will have
a lasting impact. As Rebek expressed it a few years ago in
his overview of The Skaggs Institute, "the ultimate research
identity of The Skaggs Institute will be the scientists it
produces."
Note
on the author: Ulrika Kahl is a research associate in TSRI's
Department of Neuropharmacology.
|
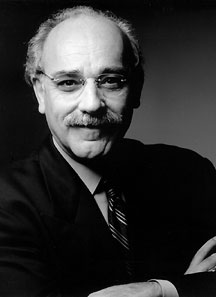
Professor Julius Rebek's most important
contributions to science to date are probably the many experimental
methods that he together with co-workers developed in self-assembling
systems and combinatorial chemistry. Photo
by Mark Dastrup.
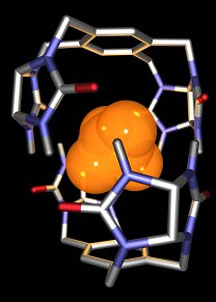
The Rebek lab's self-assembling capsuledubbed
the "tennis ball"encapsulates methane and is held
together by eight hydrogen bonds. The capsule has a lifetime
of about one second. Illustration by Arash
Rebek and Lubomir Sebo.
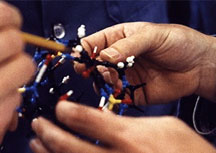
Since 1970, over 150 students and associates
have studied under Rebek's guidance. Photo
by Arash Rebek.
For more information, see:
The Rebek lab
|